(*****This series of 4 BLOG posts (1,2,3,4) is extracted from my paper from the Proceedings of the Range Beef Cow Symposium XXVI, November 18-20, 2019. Mitchell, NE. pages 37-49.*****)
The start-to-end environmental footprint – called a life cycle assessment (LCA) – of cultured meat at large scale is not available as no group has yet achieved this feat. I have tried to summarize the literature on the basis of kg of final product (Table 1), but note there are differences in a kg milk versus a kg of beef, and even a two-fold difference in the assumptions made as to the protein content of cultured meat. It is therefore close to impossible to do an apples to apples comparison. The values vary dramatically depending upon the assumptions made, and the boundaries of the LCA.
The functional unit (i.e. metric of the comparison) matters – whether kg carcass weight, kg product, kg of nutritional value (e.g., protein), and then of course the quality of that protein or food source. Changes in the functional unit (FU) alters the results quite dramatically, and therefore, the development of a FU which would reflect the complete integrative nutritional function of meat substitute is needed. It is obvious that meat substitutes have different nutritional profiles and, therefore, nutritional value. At the same time, different aspects of nutritional quality (protein and amino acid content, vitamins, fat and fatty acids, micronutrients etc.) vary in different proportion in meat substitutes. Therefore, it is necessary to develop a complex nutritional value estimate, which would reflect the qualities of meat and meat substitutes for further studies.
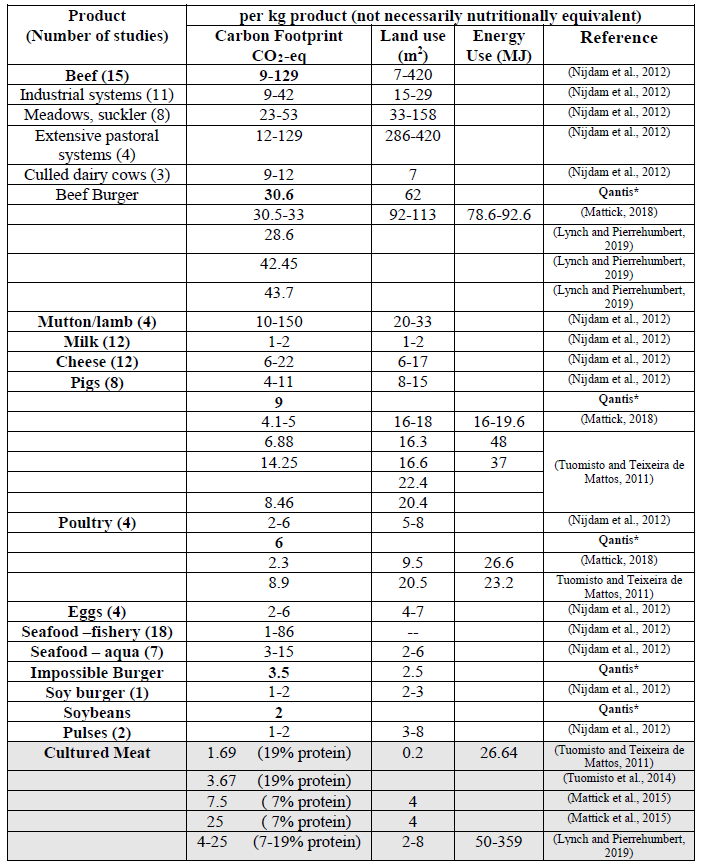
Table 1. Carbon Footprint CO2-eq, land use (m2), and energy use (MJ) per kg product for different products in a number of different studies. *Qantis (https://quantis-intl.com/).
In looking at Table 1, some general rules always apply: the carbon footprint per kg product increases as you go from one trophic level to the next (i.e. plants to animals that eat plants), and from single stomach (monogastric) animals (e.g., chickens/pigs) to ruminants (e.g., cattle and sheep). However, ruminants can eat forage that monogastrics, humans included, cannot. Ruminants consume byproducts (e.g. distiller’s grains) and crop residues (e.g. almond hulls) that would otherwise go to waste or into landfills.
Eighty-six (86%) of the global livestock feed dry matter (DM) intake consists of feed materials that are not human edible. Producing 1 kg of boneless meat requires an average of 2.8 kg human-edible feed in ruminant systems and 3.2 kg in monogastric systems (Mottet et al., 2017).
It should also be noted that land use numbers in Table 1 do not differentiate between arable land, and land that has no other human food purpose. Many ruminants graze marginal land, or crop residues, and convert that otherwise inedible forage into milk and meat. Conversely, cellular meat will require the provision of food grade nutrients supplied directly to the cells growing in the bioreactor, and waste streams will need to be disposed of following production of the cultured product. The LCA of this aspect of cellular meat remains unknown.
It is worth noting that the very favorable cultured meat LCA (Tuomisto and Teixeira de Mattos, 2011), oft-cited by proponents of cultured meat, was funded by New Harvest, a non-profit research institute accelerating breakthroughs in cellular agriculture, and has been especially criticized for assuming cultured mammalian meat will be able to be grown using cyanobacteria hydrolysate as the nutrient and energy source for muscle cell growth, as this medium is more commonly used for yeast cells; and for ignoring the environmental impacts of growth factors and vitamins, as the cells cannot grow without these supplements and they are both difficult to isolate and synthesize.
The functional unit (FU) of the alternative meat discussion to date has been, oddly enough, hamburger patties, ground beef.
As mentioned previously, the FU used as the comparator can dramatically alter the sustainability metrics of any system (lb carcass weight is different to lb edible meat, and lb protein, and lb of animal source food) – especially if the comparators differ nutritionally.
An average weight steer (1,300 lb) produces an 806 lb carcass which yields 639 lb edible beef, of which 38% is ground beef (i.e. 62% is not!). Primal cuts are obviously more valuable than ground beef, and the by-products of beef (including hides, offal, blood, tallow, bones, and bone meal) which represent approximately 11.7% of the value of a carcass are not factored into many LCA analyses. There are also offal products such as beef tongue and tripe, favorites of many ethnic communities, which are unlikely to be replicated via cell culture technology.
According to the FAO, global animal agriculture is estimated to account for 14.5% of anthropogenic GHG emissions which can be broken down into beef (5.9%), cattle milk (2.9%), pork (1.3%), buffalo milk and meat (1.2%), chicken meat and(1.2%), and small ruminant milk and meat (0.9%) (FAO).
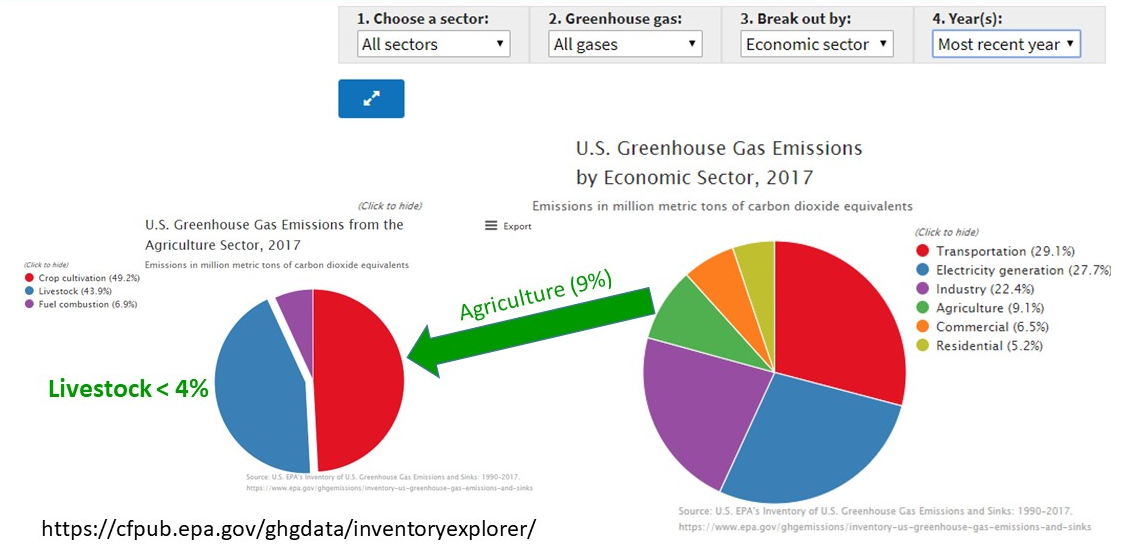
Nationally agriculture accounts for 9% emissions, slightly less than 4% is animal agriculture. https://cfpub.epa.gov/ghgdata/inventoryexplorer/
In the United States, all of agriculture is responsible for 9% of the US GHG emissions (US EPA). Fossil fuel-based energy is responsible for over 80% of total US GHG emissions, as compared to slightly less than 4% from animal agriculture. To put this in perspective, it has been estimated that eliminating ALL of US animal agriculture would decrease US GHG by 2.6%, but would also create a food supply incapable of supporting the US population’s nutritional requirements .
LCA REFERENCES
Lynch, J. and R. Pierrehumbert. 2019. Climate Impacts of Cultured Meat and Beef Cattle. Frontiers in Sustainable Food Systems 3(5).
Mattick, C. S. 2018. Cellular agriculture: The coming revolution in food production. Bulletin of the Atomic Scientists 74(1):32-35.
Mattick, C. S., A. E. Landis, B. R. Allenby, and N. J. Genovese. 2015. Anticipatory Life Cycle Analysis of In Vitro Biomass Cultivation for Cultured Meat Production in the United States. Environ Sci Technol 49(19):11941-11949.
Mottet, A., C. de Haan, A. Falcucci, G. Tempio, C. Opio, and P. Gerber. 2017. Livestock: On our plates or eating at our table? A new analysis of the feed/food debate. Global Food Security 14:1-8.
Nijdam, D., T. Rood, and H. Westhoek. 2012. The price of protein: Review of land use and carbon footprints from life cycle assessments of animal food products and their substitutes. Food Policy 37(6):760-770.
Tuomisto, H. L., M. J. Ellis, and P. Haastrup. 2014. Environmental impacts of cultured meat: alternative production scenarios. Pages 8-10 in Proc. Proceedings of the 9th international conference on life cycle assessment in the agri-food sector.
Tuomisto, H. L. and M. J. Teixeira de Mattos. 2011. Environmental Impacts of Cultured Meat Production. Environmental Science & Technology 45(14):6117-6123.